A Trillion Rogue Planets and Not One Sun to Shine on Them
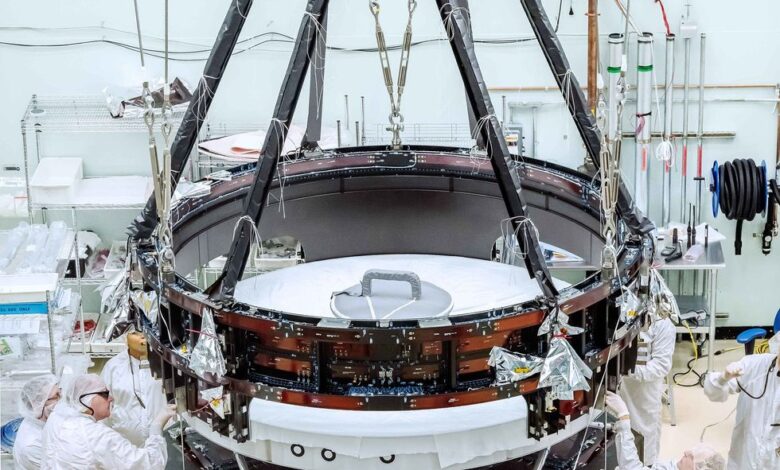
On 29 October 2020, astronomer Przemek Mróz from the University of Warsaw and an international group of collaborators reported a peculiar flicker of light originating from halfway across our galaxy. The signal, designated OGLE-2016-BLG-1928, was extremely subtle. It caused a single star to brighten and dim by about 20 percent over a 6-hour period, just once, never repeated. But the implication of that little flicker was huge: It was the first credible sighting of an Earth-size “rogue planet,” a world untethered to any star, floating freely between the stars.
“It’s always exciting when you find a truly new thing. This is why I became a scientist,” Mróz says. And, oh boy, did he get what he wanted.
Over the previous decade, three independent sky-monitoring projects had found evidence of massive, Jupiter-like planets drifting alone through space. OGLE-2016-BLG-1928 was the first hint that Earth-size free-floating planets are out there, too. Last year, a group working on the MOA (
Microlensing Observations in Astrophysics) survey found MOA-9y-5919, a second likely rogue Earth. Put these discoveries together, and you get not just a few oddities, but an entire, previously unknown class of celestial objects. Soon we will know a lot more: Two upcoming space telescopes scheduled for launch by the United States and China will track down these wanderers and unlock vital information about them, using fast infrared cameras.
“The conclusion is now strong. We have a huge population of low-mass, free-floating planets in the Milky Way,” Mróz says. “They seem to be really common. Current estimates are that there may be seven such planets per every star.” That translates to potentially trillions of rogue planets in our galaxy alone. We just didn’t know about them until now.
Chris Philpot
Not only do rogue planets outnumber visible stars, they probably also outnumber conventional planets like Earth, the ones that orbit their own suns and bask happily in their warmth. If anything, worlds like ours are the outliers. The tremendous abundance of the rogues implies that the process of planet formation is extremely messy, with many worlds getting kicked into the void almost as soon as they take shape. Lots of potentially habitable planets probably end up cold and desolate as a result. Then again, some exobiologists, who search for life outside Earth, speculate that certain types of rogue planets could become roving ambassadors, ferrying life across interstellar space.
The discovery of free-floating, rogue planets also highlights how technology is enabling us to see into previously hidden parts of the universe. These objects emit no light and cast no shadows. They are impossible to observe directly, notes astronomer and planet hunter
Scott Gaudi of Ohio State University. Scientists can only “feel” them by the way their gravity bends light. The gravitational pull of a planet can act as a magnifying glass, briefly amplifying the light from more distant stars, as happened with OGLE-2016-BLG-1928. This phenomenon, called gravitational microlensing, is tricky to detect but uniquely revealing. “There’s no way we would know these planets were there except for microlensing—it’s the only way to do it,” Gaudi says.
Since their crude beginnings in the early 1990s, microlensing surveys have steadily improved as astronomers have equipped their telescopes with larger CCD (charge-coupled device) sensors, faster electronics, and more efficient software to filter out false alarms. The efforts so far have yielded fewer than 10 likely free-floating planets —but
NASA’s US $3.9 billion Nancy Grace Roman Space Telescope, set for launch in 2027, promises to revolutionize the field. Along with instruments to study exoplanets that orbit distant stars, it will conduct the first dedicated microlensing survey from above Earth’s distorting atmosphere, using a 2.4-meter light-collecting mirror and state-of-the-art infrared detectors to scan wide swaths of the sky. By 2032, Roman could increase the number of known rogue planets by a factor of 100.
The Roman Space Telescope will conduct the first comprehensive census of our galaxy’s rogue planets.Goddard Space Flight Center/NASA
Roman’s data will be blended with observations from other space- and ground-based observatories, most notably Earth 2.0, a complementary space telescope being developed by the Chinese government and aiming for a 2028 launch. The result will be the first broad census of our galaxy’s untethered worlds. This should be a high point for exoplanet research, and yet there’s a wrinkle. Data from Roman will be immediately available to the worldwide scientific community, but Earth 2.0’s data will probably remain proprietary for a time. Unless NASA builds its own version of Earth 2.0—a mission that now exists only at the concept stage—Chinese astronomers and their collaborators who have access to both Earth 2.0 and Roman could end up scooping the rest of the astronomical world.
A New Take on How Planets Form
Few things in science are as exciting as overthrowing the conventional wisdom, and rogue planets are doing a bang-up job in that regard. Back in 1734, the Swedish inventor and natural philosopher
Emmanuel Swedenborg proposed that Earth and the other planets had formed from a nebulous cloud surrounding the infant sun. Over the years, this “nebular hypothesis” went through many modifications, incorporating new insights about gravity, turbulence, and atomic behavior. But the core concept survived: The solar system evolved steadily from disorder to order, with the planets emerging in their current arrangement around the sun.
That lovely picture of harmony began to crack in 1995, when Swiss astronomers
Marcel Mayor and Didier Queloz discovered 51 Pegasi, the first known planet around another, sunlike star. The world they found contradicted many of the standard ideas about how planets are born. About half the mass of Jupiter, it orbits much closer to its star than any planet in our solar system, in a zone where temperatures are far too high for any planet to form. Soon after, researchers found similar “hot Jupiters” around other stars.
The discovery of 51 Pegasi in 1995, a Jupiter-size planet orbiting very close to its star, upended centuries-old beliefs about how planets form.
The only way such extreme worlds could exist, scientists concluded, is if they had formed much farther out and then migrated inward during a high-drama stage of chaos and instability.
Sean Raymond, who studies planetary formation and evolution at Laboratoire d’Astrophysique de Bordeaux in France, regards the discovery of hot Jupiters as a moment of astronomical awakening. “It really broadened our minds in terms of how planets form. It showed us that they don’t have to stay put. They can move around, they can get kicked into weird orbits,” he says. As the number of detected planets has swelled to more than 5,600, astronomers have continued to find more and more oddballs: not only hot Jupiters, but also worlds that orbit sideways, in retrograde orbits, or at huge distances from their stars. If planets could be kicked into such extreme orbits, then perhaps they could get kicked out of their systems entirely.
Researchers like Raymond and
Alessandro Morbidelli at Observatoire de la Côte d’Azur in France began reexamining our solar system, realizing that it bears the scars of its own early instability. For instance, Uranus is tipped on its side, and Neptune is also oddly askew. The current best explanation, Raymond says, is that there were a whole bunch of planetary embryos flying around willy-nilly in the early solar system. Some collided violently to form Uranus and Neptune. At least one Neptune-size world probably got spit out into interstellar space, along with many smaller objects. “It’s totally plausible for planetary embryos the size of Mars or even the Earth to get ejected,” Raymond says. The discovery of rogue planets backs up those models.
Then in October 2017, astronomers at the
Haleakalā High Altitude Observatory in Hawaii discovered ‘Oumuamua. The first interstellar object observed passing through our solar system, it affirmed this chaotic view of planetary formation. ‘Oumuamua appears to be a comet or asteroid-like fragment that was born around another star and then cast out into interstellar space—a miniature version of a rogue planet. “Seeing ‘Oumuamua implies that there’s a lot of these smaller free-floating things out there,” Gaudi says.
The 2017 discovery of the interstellar object ‘Oumuamua [circled in blue] provided direct evidence that planet formation can send objects into exile.K. Meech et al./ESO
Rogue planets are shaking up astronomers’ ideas about planetary formation in another way, too. At least some of the rogues appear to have formed in place: not exiled but born in solitude, apart from any star. These are the only type of rogue planets that can be observed directly.
That’s because planets that are young enough and massive enough emit enough heat to be detected with an infrared telescope. In 2000, competing
British and Spanish teams found a population of these massive, starless, newborn planets wandering around a stellar nursery in Orion. Last year, another group used the James Webb Space Telescope to zero in on the Orion Nebula, where they found 540 more of these self-made planets.
The only way to understand the full complexities of planet formation—which ones get tossed, which ones survive, which ones are born on their own—is to get good statistics on what is out there. And the only way to get better statistics on what’s out there is to peer into the pure darkness.
And the only way to see planets in that darkness is with gravitational microlensing.
The Hunt for Rogue Planets Begins
The goal of using gravity to see into the dark has inspired three major, long-running searches. In 1992, Andrzej Udalski, an astrophysicist at the University of Warsaw, established the first of these surveys. OGLE, the
Optical Gravitational Lensing Experiment, began scanning the sky using a 1-meter telescope and a then-state-of-the-art single-chip CCD detector. It’s been running continuously ever since but has graduated to a 1.3-meter telescope at Las Campanas Observatory in Chile, now working with a 32-chip mosaic CCD detector that offers much better resolution and a wider field of view. “I’m one year younger than the project itself,” jokes Mróz, who joined OGLE in 2010, shortly after it entered its fourth and newest configuration.
Three years after OGLE’s founding, Yasushi Muraki of Nagoya University in Japan and Philip Yock at the University of Auckland in New Zealand launched
MOA. Like OGLE, MOA has been repeatedly upgraded, with detector improvements and a switch from a 0.6-meter telescope to a telescope three times as large situated at Mount John University Observatory in New Zealand.
The Korea Microlensing Telescope Network’s 1.6-meter telescope in Chile is one of three that KMTNet uses to search for rogue planets using gravitational microlensing.B. Tafreshi/CTIO/NOIRLab/NSF/AURA
Most recently, in 2009, the Korea Astronomy and Space Science Institute inaugurated KMTnet (
Korea Microlensing Telescope Network), which is using three 1.6-meter telescopes at the Cerro-Tololo Inter-American Observatory in Chile, the Siding Spring Observatory in Australia, and the South African Astronomical Observatory in the Karoo region. Having three telescopes distributed around the world gives KMTnet the ability to watch the sky 24/7.
In 2023, a group working on the MOA (Microlensing Observations in Astrophysics) survey used this 1.8-meter telescope in New Zealand to find MOA-9y-5919, a second likely rogue Earth.
Dave Smith/Flickr
Although these projects differ in their technical approaches, they all follow a similar science brief. They build on an effect of general relativity that Albert Einstein described in a 1936 paper in
Science, “Lens-Like Action of a Star by the Deviation of Light in the Gravitational Field.” Einstein had already established that the gravitational field of a massive object can deflect the path of a beam of light; the observation of bent starlight during the 1919 solar eclipse validated his theory of general relativity and turned Einstein into a global celebrity.
What Einstein described in his 1936 paper was a more subtle phenomenon: If a massive object happens to be aligned almost exactly with a more distant star, the object’s gravity will warp and distort the star’s light. Stars are much too far away for us to observe the shape of the distortion, though, and Einstein dismissed the significance of his own finding. “Of course, there is no hope of observing this phenomenon directly,” he wrote. One thing he hadn’t considered is that the lenslike effect that warps the image of the star also amplifies its brightness. And one thing he couldn’t have known was that someday it would be possible to monitor millions of stars at once to look for the occasional flicker caused by the chance alignment between a star and a more nearby object.
How Gravitational Microlensing Works
Most rogue planets are far too faint to be observed directly. But when a rogue planet happens to pass directly in front of a distant star, the planet’s gravity warps and distorts the star’s light and amplifies its brightness for a brief time. The Roman Space Telescope will look for those rare and fleeting events.
To date, the strongest microlensing event of an Earth-size planet produced only a doubling of brightness over a few hours. To catch such subtle signals among a vast field of stars, microlensing surveys use software to send an alert whenever a notable brightness change is detected. Then a human observer can start watching the event as it unfolds and request follow-up observations.
Basically, modern microlensing surveys rely on playing the odds. At any one moment, the likelihood of any one planet happening to pass right in front of a more distant star is minuscule. “Just one in 100,000 stars is microlensed at a time, so you have to observe very dense regions of the sky,” Mróz explains. “If you want to find a planet, you have to observe millions and millions of stars on a time scale of several minutes at least several times per hour.”
The OGLE, MOA, and KMTnet teams converged on a technique for detecting these rare events. They aim their telescopes toward the constellation Sagittarius, which happens to lie in the direction of the dense center of our galaxy—the galactic bulge—where about 400 million detectable stars are packed tightly together. Then they wait, as the orbital motion of objects within the Milky Way causes planets and stars and everything else to drift by. And they watch for any telltale changes in the brightness of one star among the multitude due to a planet passing in front of it, briefly magnifying its light.
Microlensing a Rogue Planet
www.youtube.com
Before OGLE, nobody had dared attempt the delicate task of spotting microlensing events, and there were a lot of ways to make mistakes as it and the other surveys got underway. “We recognized that there could be false alarms,” says
David Bennett, a veteran member of the MOA team who works at NASA’s Goddard Space Flight Center. He lists some of the potential errors: Atmospheric distortion can create illusory brightness changes, some naturally variable stars can mimic a lensing event, and a fast-moving massive object like a brown dwarf can mimic a slower-moving planet.
Many microlensing events go unnoticed, Bennett adds, because telescopes on Earth can’t watch the same stars around the clock. And even when you do see them, the signals from planet-size objects are relatively weak and faint. The strongest microlensing event of an Earth-size planet produced just a doubling of brightness over a few hours. All of the microlensing surveys have therefore set up automated triggers. When the software detects a brightness change that looks interesting, it sends out a notice. Then a human observer can start watching the event as it unfolds and request more detailed observations.
Pushing through all these obstacles, the MOA and OGLE teams jointly detected the first-ever microlensed planet in 2003,
announced a year later as OGLE 2003-BLG-235/MOA 2003-BLG-53. (The detections are cataloged as events rather than as objects, hence the tortured nomenclature.) The planet in question was massive, about 1.5 times as hefty as Jupiter, and it was not a free floater. Planets in conventional orbits are much easier to find because their host stars produce a much stronger lensing signal. Still, this was a landmark event, proof that it was possible to detect a planet by gravity alone.
The galactic bulge lies at the center of the Milky Way and contains some 400 million visible stars. In the hunt for rogue planets, the Roman Space Telescope, along with the ground-based surveys OGLE, MOA, and KMTNet, will be trained on this densely starry area.S. Brunier/ESO and J. Skowron/OGLE
Then came another long slog through the data, to track down an actual rogue planet between the stars. A 2011
report, jointly published by the OGLE and MOA teams, presented the first evidence for bona fide planetary rogues, but with significant uncertainties. It took another nine years for Mróz and his OGLE colleagues to sift through observations of 50 million stars and 2,617 recorded microlensing events and to present, at long last, the well-supported event of OGLE-2016-BLG-1928, the first Earth-size rogue.
By the time the OGLE team made its 2020 announcement, there were at least eight plausible sightings of other, more massive free-floating worlds. Reassuringly, the researchers working on OGLE, MOA, and KMTnet all arrived at the same basic results.
How to Detect Gravitational Microlensing
Knowing that rogue planets exist was just the prerequisite for asking the really juicy scientific questions: Exactly how many rogues are there? Where did they come from? And what are these lonely worlds actually like? When you’re talking about planets that are cloaked in eternal darkness, seen only by virtue of their gravitational pulls, getting answers is not so easy. The only way to make progress is by calling on the two key resources that every researcher clamors for: more data and better technology.
Start with the seemingly simple question of the total number of rogue planets. The OGLE team ran detailed statistical analyses of their data to come up with the estimate of about a trillion free-floating planets in the Milky Way. But that number is built on a tiny sample. And some of the specimens in that sample may actually be planets that are orbiting their suns at such a great distance (equivalent to a bit beyond Pluto in our solar system) that they look like rogues.
Fortunately, scientists already have a fabulous piece of equipment for settling this issue: the mighty
James Webb Space Telescope, launched in 2021. Using its powerful infrared vision, JWST can readily resolve individual stars in the galactic bulge, aim at the location of a reported rogue planet, and look for a stellar parent nearby. Bennett says he’s submitted a proposal to JWST to look at seven of the presumed free-floating planets.
The Roman Space Telescope will have a much wider field of view than NASA’s other giant space telescopes. This image shows millions of simulated galaxies; the many areas that Roman will be able to image in a single snapshot are outlined in yellow, dwarfing the Hubble Space Telescope’s field of view, outlined in white. A. Yung/Goddard Space Flight Center/NASA
But there are a lot of astronomers and a lot of projects competing for JWST’s time, and Bennett hasn’t yet received an approval. Ground-based telescopes, like the W.M. Keck Observatory and Subaru Telescope, both in Hawaii, can help with the identity check, but they are not nearly as sensitive or precise as JWST.
The dream tool for exploring free-floating planets would be a telescope that watches the galactic bulge from space, where the views are crystal clear; observes stars in infrared light, which pierces through the interstellar dust in our galaxy; has a wide field of view, to take in millions of stars at once; and attentively measures the brightness of the stars for long periods of time, to make sure we don’t miss any fleeting microlensing events caused by passing rogue planets. It’s quite a wish list. The Hubble Space Telescope, launched in 1990, is hopeless for rapid observations in the infrared. JWST, like most of today’s most powerful observatories, is designed to look narrow and deep, which is terrible for a large-scale survey.
The upcoming Roman Space Telescope, on the other hand, ticks all the boxes. “It is just an ideal machine to detect very low-mass free-floating planets,” Gaudi says.
The Roman Space Telescope is ideal for observing the faint signals produced by rogue planets. It will observe primarily in infrared light, and its wide field of view will take in millions of stars at once.Chris Philpot
Melissa Vess, spacecraft systems engineer for the Roman, brags like a proud parent as she reviews the telescope currently taking shape at NASA’s Goddard Space Flight Center in Greenbelt, Md. Roman’s 2.4-meter mirror is the same size as the one in Hubble—no coincidence, since they were both built to the same basic specs as mirrors used in old KH-11 spy satellites, launched by the U.S. National Reconnaissance Office from 1976 to 1990. But Roman’s capabilities are starkly different. It has much faster optics (that is, a much shorter focal length relative to its diameter), which is part of the reason why each Roman image will cover about 100 times as much sky as a Hubble image. So: clear view and wide field, check.
The Roman Space Telescope’s 2.4-meter mirror is based on a spare left over from the KH-11 spy satellites, which were launched from 1976 to 1990.Chris Gunn/NASA
Roman’s imaging and spectroscopy camera, the
Wide Field Instrument, incorporates 18 of the latest 4K-by-4K-pixel H4RG-10 detectors from Teledyne, each detector providing 16.8 million pixels of sky coverage. It’s “an evolution of what’s on JWST, and well beyond what Hubble can do,” Vess notes. The instrument is currently undergoing environmental testing at Ball Aerospace in Broomfield, Colo. The detectors are sensitive to colors from green-blue to red, but their real strength is going beyond the visible-light spectrum into the infrared, out to a wavelength of 2.3 micrometers. With just 55 seconds of exposure time, Roman will be able to detect sources about a 40-millionth the brightness of what the human eye can see. And the instrument will refresh its view of the galactic bulge every 15 to 20 minutes, quickly enough to catch the blippy microlensing signals of planets smaller than Earth, smaller than Mars even. So: fast and attentive, check.
Roman’s element wheel, a key part of the Wide Field Instrument, will tune the wavelengths of light that reach the detectors from astronomical objects and spread the light into telltale spectra.Ball Aerospace
Not only will Roman be able to reach deeper into the infrared than Hubble can, it will also provide a much more steady view of the heavens. Hubble is stuck in low Earth orbit, meaning that every 45 minutes it dives in and out of Earth’s shadow. The rapid heating and cooling of the telescope causes Hubble’s structure to flex. But Roman, like JWST, will orbit in steady sunshine around L2, a gravitational balancing point 1.5 million kilometers from the sun. With no wild thermal swings to worry about, engineers at L3Harris in Melbourne, Fla., were able to design a more stable structure for Roman. The telescope’s 4,000 triple-junction solar cells, which can harvest a wider range of wavelengths than commercial solar cells can, never plunge into darkness, so they’ll generate at least 4.1 kilowatts of power while shielding the rest of the instrument from light and heat.
Roman’s solar array will generate at least 4.1 kilowatts of power while simultaneously shielding the rest of the instrument from light and heat.Jolearra Tshiteya/NASA
About half of that power will be used by Roman’s hundreds of temperature sensors and its heaters, which will keep the telescope’s interior thermally stable to within about 0.1 °C. Meanwhile, a two-stage radiator and metal thermal straps will chill the Wide Field Instrument’s detectors to an optimal −185 °C, so that heat from the electronics doesn’t overwhelm the infrared signals from starlight. Roman has another suite of radiators and thermal straps to chill its other major instrument: an experimental device called the Coronagraph, which will blot out starlight to reveal exoplanets nestled right beside their stars (although it’ll be useless for finding rogue planets in the dark).
Each of the 18 CCD detectors in Roman’s Wide Field Instrument can capture more than 16 million pixels of visible and infrared light emitted by astronomical objects, and then convert the light into electrical signals. Chris Gunn/NASA
As a do-it-all telescope that will look both wide and deep into the universe, the Roman Space Telescope will return a torrent of data. It is expected to transmit 1.4 terabytes of data each day, more than 20 times the data flow of JWST and more than 500 times the rate for Hubble. To manage that information firehose, Roman carries a hefty 1.7-meter high-gain antenna, which will link to Earth via both S-band and Ka-band radio: the lower bandwidth S-band for command and control, and the higher bandwidth Ka-band for science data. The antenna can downlink 500 megabits per second, but “the pipe in is bigger than the pipe out,” Vess notes: At peak moments, Roman’s Wide Field Instrument can deliver around 2.5 gigabits of data per second. To avoid data logjams, the observatory will carry a pair of flash-memory recorders developed at NASA Goddard, each of which can store 9 terabytes of data as needed.
The 1.7-meter high-gain antenna on Roman will link to Earth via both S-band, for command and control, and Ka-band radio, for science data, at downlink speeds of up to 500 megabits per second.Chris Gunn/NASA
The more serious bottleneck in Roman’s data pipeline will probably be here on Earth. The 18-meter radio dish in White Sands, N.M., that will serve as Roman’s primary receiving station won’t be able to keep up with the space telescope’s maximum transmission capabilities. Vess says that other ground stations—the European Space Agency’s upcoming 35-meter antenna for
NNO-3 in Western Australia and the Japanese Aerospace Exploration Agency’s new 54-meter antenna for the Misasa Deep Space Station in Japan’s Nagano prefecture—should do better.
Astronomers’ plans to unleash Roman as the world’s greatest rogue-planet-hunting machine are even more impressive given that microlensing was barely considered a viable technique when the telescope project began in 2011. Now the search for gravitational blips from planets as well as black holes is part of Roman’s core mission. Thirty years of searching from the ground has turned up about 10 likely free-floating candidates. According to a recent
paper by Bennett and other members of the MOA team, in the first five years after its 2027 launch, Roman is expected to discover about 1,000 of them. “That’s probably a lower limit,” Bennett adds dryly.
Geopolitics Comes for Astronomers
As capable as it is, the Roman Space Telescope will still need an assist from other observatories. One of the vexing realities of rogue-planet science is that a single observation of a microlensing event provides incomplete information. Was the object that caused the brightness spike a fast-moving massive planet, or a slow-moving lightweight one? In most cases, there will be no clean way to tell from the Roman observations alone. Multiple solutions will fit equally well.
OGLE, MOA, and KMTnet will watch the same patch of sky and attempt to complement the data from Roman. So will an upcoming South Africa–based microlensing project called
PRIME (PRime-focus Infrared Microlensing Experiment), which is designed to work in collaboration with Roman, using similar detectors. These various earthbound observatories will be limited in how much they can help, however. “Most of the Roman free-floating planet microlensing events will not have any detectable signal from the ground,” says Weicheng Zang of the Harvard-Smithsonian Center for Astrophysics and Tsinghua University in Beijing.
Roman’s observations need outside corroboration to accurately estimate planetary mass. Such mass estimates are crucial for understanding rogue planets. If you know the masses of the rogues, you can test theories of planet formation and estimate how many potential Earths get cast aside by their stars. You can investigate whether some planets really do form in place. You can explore the types of environments that may exist on rogue planets and whether some of them could even support life. If you don’t know the masses…you can’t.
Inside the Hunt for Rogue Planets
Project
Lead organization
Start date
Status
Optical Gravitational Lensing Experiment (OGLE)
University of Warsaw
1992
Operates a 1.3-meter telescope in Chile. In 2020, announced the first discovery of an Earth-size free-floating planet.
Microlensing Observations in Astrophysics (MOA)
Nagoya University and University of Auckland
1995
Operates a 1.8-meter telescope in New Zealand. In 2023, announced the second discovery of an Earth-size rogue.
Korea Microlensing Telescope Network (KMTNet)
Korea Astronomy and Space Science Institute
2009
Operates three 1.6-meter telescopes in the Southern Hemisphere for 24/7 sky coverage.
PRime-focus Infrared Microlensing Experiment (PRIME)
Osaka University
2025 (scheduled)
1.8-meter near-infrared telescope at South Africa’s Sutherland Observatory, designed to complement the Roman Space Telescope.
Nancy Grace Roman Space Telescope
NASA
2027 (scheduled)
Will carry a 2.4-meter mirror and advanced infrared detectors to conduct wide-field surveys of the galactic bulge.
Earth 2.0 telescope
Chinese Academy of Sciences
2028 (scheduled)
One of the seven telescopes of this space-based observatory will complement Roman’s observations. Under development in China.
Contemporaneous Lensing Parallax and Autonomous Transient Assay (CLEoPATRA) telescope
NASA
Unknown
A proposed 50-centimeter space-based telescope to complement Roman, now awaiting NASA approval for a full study.
Zang recognized the problem three years ago and devised a clever solution: a second, much smaller space telescope, the aforementioned Earth 2.0, which would watch the same stars as Roman but from a vantage hundreds of thousands of kilometers away. (The Earth 2.0 design also includes six additional telescopes to study ordinary, star-bound planets passing in front of their stars in a different patch of sky.) When the same rogue planet is observed from two such widely separated locations, the brightness increase due to lensing shows up at different times. In essence, the two observers get different perspectives on the shape of the gravitational distortion created by the planet. When you compare the timings and combine those perspectives, you then have enough information to nail down the planet’s mass. With Earth 2.0 added into the mix, Zang says, “we will get 200 free-floating planets with good mass estimates.” Relying only on ground-based telescopes, he says, you’d get mass estimates for just 10 rogue planets.
In this division of labor, Roman does the heavy lifting by getting the high-resolution images of the microlensing events. Earth 2.0 needs to be only powerful enough to measure the total brightness of the microlens as seen from its location. As a result, a small, 35-centimeter telescope and a spacecraft built with a more modest budget—hundreds of millions rather than billions of dollars—should be sufficient to do the job. Earth 2.0 was approved by the Chinese government last year and is now on track to launch just after Roman, in 2028.
OGLE’s telescope in Chile captured these images of area 534 of the galactic bulge. To find a rogue planet, you need to spot the single, brief flicker among all of those stars. Szymon Kozlowski/OGLE
One scientific problem closed, but one political problem opened. Data from Earth 2.0 will initially be available only to researchers using the telescope. Data from Roman, however, will be immediately accessible to the global astronomy community.
“The Roman Space Telescope is based on the principle of open science. And I am front and center in helping scientists to be more intellectually generous,” says
Richard Barry of NASA Goddard, who is a member of both MOA and the Roman microlensing team. As a federal employee, he and other NASA staff members are barred by U.S. rules from joining any Earth 2.0 collaboration. “There’s a number of university colleagues of mine who can work with the Chinese team,” Barry says. “But nobody from NASA [can]. I’m not allowed to even talk to folks [from China] without having an escort or having to be debriefed.”
Barry is worried. “China will have a proprietary period on their Earth 2.0 data that is long enough to where they will simply scoop all of the mass measurements right out of the data,” he says. “This feels to me like a slap in the face. It’s like we’re being punished for being open.”
The Roman Space Telescope will orbit at L2, about 1.5 million kilometers from the sun, as will China’s Earth 2.0 telescope and NASA’s proposed CLEoPATRA. NASA
I put the question to Zang: Is there any possibility that Earth 2.0 data could be open access as well, so that everyone could work on mass measurements together? “Yes, this is an issue,” he says after a pause. “The Earth 2.0 members and collaborators can access the data first. Then the data will be public to anyone, although currently I don’t know how long is the delay. I myself would like to make the data public as early as possible.” Compounding the issue, he adds, is that China has “many” grad students working on gravitational microlensing, whereas the United States has “less than 10.” Those grad students will be crucial for sifting through the data that comes back from Roman and Earth 2.0.
Barry is now working hard to build an American, open-access version of Earth 2.0. His proposed space telescope is called CLEoPATRA (a tortured backronym from
Contemporaneous Lensing Parallax and Autonomous Transient Assay). It would operate much like Earth 2.0, using a 50-centimeter telescope to monitor the brightness of microlensing events while looping around L2, up to 800,000 kilometers away from Roman. Barry pegs the cost of the mission at about $40 million, or about 1 percent of the Roman budget. He’s currently awaiting a decision on whether NASA will commission a full study of CLEoPATRA, a prelude to a formal proposal to fly.
The 54-meter antenna at Japan’s Misasa Deep Space Station will help download the 1.4 terabytes of data that Roman is expected to collect each day.GREAT Project/JAXA
There are other possible workarounds for researchers who don’t have access to Earth 2.0. The European Space Agency’s
Euclid space telescope, which just began operating near L2, might be able to take breaks from its primary mission to provide crucial, second-perspective data on the Roman observations. And new ground-based telescopes, most notably the powerful Vera C. Rubin Observatory nearing completion in Chile, may be able to coordinate effectively with Roman.
Space-based observatories can offer sharper and deeper views, such as this image of the Horsehead Nebula taken by the Euclid space telescope. Here, young and massive free-floating planets can be seen directly, without the need for gravitational microlensing. J.-C. Cuillandre/CEA Paris-Saclay and G. Anselmi/Euclid Consortium/NASA/ESA
In Barry’s dream scenario, Roman will scoop up data, CLEoPATRA will fly, Earth 2.0 will add a valuable third perspective, and scientists will get the best-possible measurements of hundreds of rogue planets across the Milky Way. Then we’ll have it all: masses, statistics, and for the first time, a complete overview of all the different types of planets that exist.
Regardless of how things pan out, NASA’s next great observatory will be a uniquely powerful explorer of the hidden universe. “Roman is going to show us
everything from 30-solar-mass black holes to free-floating planets as small as Jupiter’s moon Ganymede,” Ohio State’s Gaudi says. “It’s going to be incredible.”
IEEE Spectrum