How to Build a Power Grid on the Moon
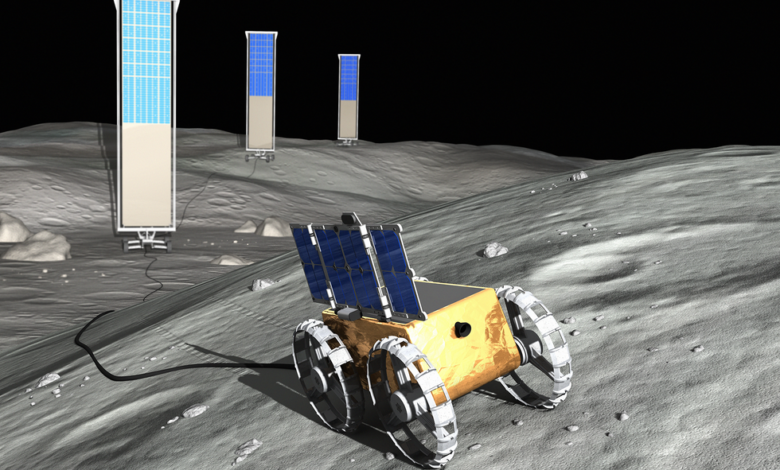
The early forays to the moon employed throwaway technologies, designed to function during the two-week period of lunar daylight, then to perish in the freeze of the equally long night. But to sustain a colony, everything must be made to survive deathly extremes. During daytime, lunar temperatures can reach 120 °C at the equator. At night the temperature falls to -220 °C, as cold as Pluto. Without a heater, equipment breaks down. Structural components grow brittle and can crack from the mechanical stresses of this huge temperature swing. Without sunlight, solar panels become useless, their broad expanse then serving only to lose heat. Batteries lose capacity, and their liquid components freeze, requiring a power-hungry cycle of using much of the power in batteries just to heat themselves.
On its way to the moon, a spacecraft also faces extreme temperature swings, with the shadowed side hundreds of degrees colder than the sunny side. A combination of insulation, reflection, and energy produced from solar panels works to keep the entire spacecraft within an acceptable temperature range throughout its flight. It’s only after the spacecraft lands that the countdown to those very challenging lunar nighttime conditions begins.
Most deep-space missions, like Voyager and New Horizons, and long-term lunar surface probes, like the Apollo Lunar Surface Experiment Package (ALSEP), survive with limited sunlight by employing radioisotope thermal generators. These generators can consistently produce heat for decades, but due to the highly radioactive fuel required, they present challenges of safety, sourcing, and regulatory approval. Solar power would be a better solution if it could serve the extremes of the lunar surface.
We and our colleagues at Astrobotic, a small Pittsburgh-based business founded in 2007, are on a mission to make space accessible to the world. The company is best known for its fleet of lunar landers, which includes Peregrine, whose first mission is scheduled for 2023, and Griffin, which is scheduled to transport NASA’s Volatiles Investigating Polar Exploration Rover (VIPER) to the lunar south pole in 2024. Now the company means to solve the power problem on the moon with a service that can collect solar energy at the poles, where sunlight is never far away, and distribute it to places sunk in darkness.
The system we intend to build on the moon, dubbed LunaGrid, will consist of a network of solar-power generating stations, or nodes, connected by transmission cables. This grid is designed to deliver power where it’s needed via a fleet of robotic rovers. Astrobotic plans to demonstrate the first-generation system as early as 2026, with the first full LunaGrid becoming operational by 2028 at the lunar south pole.
Why there? Because, through its Artemis program, NASA plans to land astronauts on the moon in 2025 and to establish a permanent base near the lunar south pole by 2030. Of particular interest in this region are deep craters whose interiors lie permanently in shadow and thus remain quite cold. These natural cold traps are where water ice can be found. That ice could provide water to drink and, after undergoing electrolysis, oxygen to breathe. The other product of such electrolysis, hydrogen, along with oxygen, might one day be used to refuel rockets. The Artemis base will have to be built close to such permanently shadowed regions. Just exploring these craters will require a lot more power than that which is already required to sustain Artemis base operations. None of the Apollo missions attempted to produce enough power to survive the lunar night. Those missions were all carried out during lunar daytime so that the astronauts could work in the warmth of the sun. Fuel cells, rather than solar cells, provided power, because solar cells of the 1960s and 1970s had a prohibitively high weight-to-power ratio. But technological advances of the past few decades have now made solar panels far lighter.
A number of small-scale lunar robots, such as the United States’ ALSEP, the Soviet Union’s Lunakhod rover, and China’s recent Chang’e landers have survived over months or years using nuclear power. They achieved this by using a radioisotope heater unit, which employs radioactivity to heat things directly, and a radioisotope thermoelectric generator, which converts radioactive heat into electricity.
Fission reactors have been proposed to sustain the Artemis mission. But this technology faces the same challenges as the earlier nuclear alternatives: Any approach that involves launching radioactive payloads would have to overcome considerable safety and regulatory hurdles to launch. Astrobotic hasn’t ruled out nuclear—it has partnered with Westinghouse to develop fission reactors for a joint NASA/Department of Energy contract. But to power the early years of the Artemis base, the company expects that NASA will adopt Astrobotic’s fully solar-powered LunaGrid. Here’s how we and our colleagues are designing it to work.
A CubeRover unfolds its solar panel, then turns it to a vertical orientation. John MacNeill
A Lunar Power Grid
LunaGrid will consist of a modular network of fixed power stations and mobile charging stations. The fixed power stations will be connected by electrical cables. Such direct electrical connections are preferable to microwave- or laser-based power transmission, which involve inefficiencies and can potentially present dangers to astronauts working in the area.
The stations will be deployed near the moon’s south pole, where the sunlit region is close enough to the nighttime region to ensure that at least some of these solar stations will always provide power. And not far away are the permanently shadowed crater floors bearing water ice. Of course, LunaGrid could also work at the north pole, should there ever be a base constructed there.
On Earth, solar panels are typically installed on a horizontal or near-horizontal surface. That works fine where the sun rises high in the sky at midday. But at the lunar poles, the sun always remains close to the horizon. So to intercept the most light, solar panels need to be set up vertically.
This image of the moon shows the south pole [bottom], a place where the sun always shines and from which solar stations can therefore always supply power. NASA
The equipment we’re planning to use contains rolled-up solar panels that stand atop a rover base. These vertical solar panels, once unfurled, reach more than 20 meters above the ground. The mobile design allows the station to descend from the lander to the lunar surface, level itself, and move to a distant position, all while managing power cables that may stretch up to 2 kilometers. This approach avoids the need for astronauts or a purpose-built robotic rover to deploy this power-generating equipment.
The roll-out solar array, from the aerospace company Redwire, of Jacksonville, Fla., is currently in use on the International Space Station. The fixed power stations will employ electrodynamic solar-cell covers from NASA Kennedy Space Center, which create a force that prevents dust particles from settling on the panels. To convert power from individual stations so that it can be fed into the grid, the system uses power-handling electronics developed at NASA’s Glenn Research Center.
What the stations cannot do for themselves is distribute power to locations separated from this grid. That’s done using small robotic vehicles, called CubeRovers, which serve as the nimble hands of the LunaGrid system. These lightweight, modular robots range in weight from 4.6 to 10.6 kilograms. Each can carry about half its mass in additional payload, and it can traverse several kilometers in a single lunar day. That’s fast for space-rover technology; in contrast, the Martian rover Spirit traveled less than 10 km over more than 2,000 Earth days. The CubeRovers will use cables to connect power-hungry equipment nearby (within about 100 meters) to the grid.
One CubeRover will stay attached to each vertical solar array when it is moving to the desired destination, then connect up with whatever needs power. Think of it as a robotic extension cord. The CubeRover will provide short-range wireless charging any place in the area where something needs power. For example, the NASA In-Situ Resource Utilization Excavator could recharge via CubeRover at a shadowed dig site, instead of having to dedicate time and energy to return to a power-generating node or to a lander with solar panels.
The transmission cables that tie power-generating stations together must be able to extend at least a couple of kilometers and also to last for years. One of the main challenges is wear to the cable when it is dragged over lunar regolith—the grit on the surface of the moon. Unlike terrestrial soil, it hasn’t been weathered into smoothly rounded particles. Instead, it resembles finely shattered glass—sharp enough to shred through layers of astronaut’s boots or to destroy the vacuum seals of sample containers. And, because the dust is also electrostatically charged by ions streaming in from the sun, it will tend to get stuck to spacesuits, sneak inside the lander, and clog equipment.
Another problem is how to transfer power to the equipment that needs it. Mechanically mated electrical connections, like what you routinely plug into your wall, would tend to get clogged with dust. Also, plugging things in would require dexterity that is difficult both for robots and people in spacesuits to achieve. So Astrobotic designed a wireless charger using technology developed by WiBotic, Bosch, and the University of Washington. It doesn’t require perfect alignment—merely proximity. To be more specific, the wireless charger and receiver coils can operate separated by as much as 4 centimeters, with up to 40 degrees of angular offset.
Powering the Planets
In 2026, when the first LunaGrid node lands near the south pole of the moon, a mobile power station will emerge from the Astrobotic lander, descend to the surface, and drive up to 2 km away, spooling out a power-transmission cable as it goes. Then the station will unroll its vertical solar array, forming LunaGrid’s second node. Both nodes will generate solar power, which can be transferred between these two stations or sent to other equipment in the area via CubeRovers equipped with wireless chargers.
By 2028, additional missions with similar equipment will link to these first two stations to yet more nodes using similar power-transmission cables, forming a full-fledged power grid that can immediately serve the Artemis moon base or other equipment.
Because LunaGrid employs vertical solar arrays, it can be used wherever the sun’s elevation above the horizon is low, such as the poles of most other moons or planets. LunaGrid could also use horizontal or tilted solar arrays at lower latitudes. For moons or planets farther from the sun, the arrays would need to be larger or have more nodes, of course, but solar power could, in principle, provide a suitable source of power for space exploration even quite far from the sun.
Imagine that it is the year 2040 and that work on the moon is routine for both robots and people. Perhaps research villages will exist, not unlike those of Antarctica. And each new lunar mission, each new enterprise, can plug into an ever-expanding grid that provides power for scientific instruments, for the construction of deep-space launch vehicles, and for the production of the fuel that they will need.
IEEE Spectrum